Advantages of the Freewing Tilt-Body UAV
[Click image to download full briefing in PDF format]
Small-Area Launch/Recovery with UAVs
The trickiest part of flying any airplane, whether manned or unmanned, has always been taking off and landing -- especially landing. The invention of the airplane required the invention of the runway. Eventually, a second type of air vehicle was introduced by Igor Sikorsky, the rotary wing or helicopter. Thus the need for runways was conquered, but at the expense of a complexity and cost disadvantage that remains today. Cost in helicopter production and operations is an inescapable function of the number of moving parts.
Conventional UAV Types |
|||||
---|---|---|---|---|---|
Core Requirements for Navy & Close-in UAV |
Fixed Wing |
Rotary Wing |
|||
• Range |
+ + |
- - |
|||
• Autonomy |
+ |
- |
|||
• Cruise Speed |
+ + |
- - |
|||
• UAV Payload / Weight Ratio |
+ |
- |
|||
• Launch & Recovery |
- - |
+ |
• Recovery -- without sacrificing performance -- is the decisive factor for shipboard & Army Close-in UAV ops |
|||||
---|---|---|---|---|---|
There are two basic kinds of conventional heavier-than-air vehicles, fixed wings and rotary wings. The former are easily high-speed, efficient cruisers, but cannot easily operate from confined spaces. Rotary wings are the exact opposite. |
|||||
---|---|---|---|---|---|
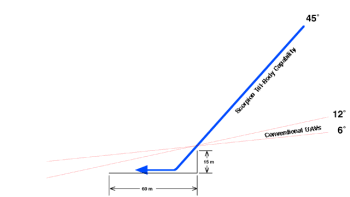
This is the 30m x 60m x 15 m "shoebox" that the US Army decreed post-Gulf War generation UAVs would have to operate from. A normal airplane glide slope is 3. Drogue chutes or flaps can steepen this to perhaps 12. But only helicopters or thrust-vectored machines like the Freewing Tilt-Body can approach at angles like 45. Fixed wings must resort to unreliable parachutes to land.
"Shoebox" Performance
In wartime one does what one must, so dangerous and destructive launch and recovery methods were employed. However, in the Lessons Learned Brief, the Pentagon insisted that the next generation of UAVs go beyond these methods. Accordingly, the UAV users created a requirement that future tactical UAVs be able to operate from a "shoebox" 30m x 60m over a 15m obstacle. Fixed-wing UAVs are clearly not meant for such a task.
Can't fixed-wing UAVs simply use parachutes to land?
This has several major disadvantages. First, before sport skydivers ever step out of their jump planes, they test the direction and intensity of winds aloft by dropping wind drift indicators and then adjusting their exit point accordingly. Soldiers have no such luxury with UAVs. They may know the wind's behavior at ground level, but conditions can be drastically different at an opening altitude of 200 feet or more. So it can be a "crap shoot" even with guidable parafoils as to whether the UAV will drift down onto the drop zone. Also, parachutes in winds can drag UAVs to damage or destruction. Lastly, repacking or replacing chutes between missions wastes time.
Then why not helicopter UAVs?
A handful of rotary wing UAVs has been demonstrated. Few have entered production over the years, and the reasons are apparent. They are quite costly to design and produce. Their high parts count insures concomitantly high costs for operations and maintenance. Reliability also is a function of the number of moving parts critical for flight. Their inherent instability requires a robust stability enhancement system. They are sensitive to turbulence due to their large prop discs, and the exposed rotor blades are seen as a danger to personnel on the ground or deck. Compared to fixed wings, helicopters are severely limited in range, endurance and/or payload capacity. Canadair's Sentinel is an example of a rotary wing UAV that has flown in pre-production.
What about hybrid helicopter/fixed-wing vehicles (i.e. vectored thrust)?
In both manned and unmanned aircraft, there have been many attempts to combine the rotary wing and the fixed wing into one vehicle. The basic idea is to harness the thrust-producing propeller or jet to temporarily also produce lift during takeoff and/or landing. Historically, most of these have failed outright. Those that succeed, such as the Harrier jump jet or the V-22 Osprey tilt-rotor, do so only at the cost of great complexity. Moreover, all of them are inherently unstable especially during transitions (i.e. from vertical to horizontal flight).
Bell Aerospace in fact has built a miniature tilt-rotor prototype called the Eagle Eye. Its two wingtip rotors tilt forward for level flight and upward for vertical or near-vertical flight. In other words, it can vector its thrust away from the direction of flight.
** "V/STOL" = "Vertical/Short Take Off & Landing"
In this Venn diagram, fixed wing UAVs are all relatively simple, low-cost vehicles, and they have high-speed efficiencies; however it is impractical to operate them from very small spaces. Helicopters or rotary wings can do this, but they are very expensive and lack high-speed and endurance. Hybrids like the Eagle Eye tilt-rotor can fly slowly like rotary wings and fast like fixed wings, but are by far the most expensive and complicated of all.
Of all the vehicles of which the Company is aware, only the Freewing™ Tilt-Body shares both Venn attributes. And beyond this, it has several important traits not shared by any of the others: it neutralizes most turbulence, providing an inherently steady sensor platform plus allowing it to fly when fixed wings are grounded; it is stall-proof and spin-free, thus improving attrition rates; it is up to an order of magnitude less sensitive to C.G. shifts, allowing flexible payloading; etc.
Freewing Tilt-Body vs. Tilt-Rotor UAVs
It is clear that both fixed wing and rotary wing UAVs suffer serious drawbacks for practical use. A vehicle capable of thrust-vectoring, or slowing down to helicopter-like speeds, is needed to break out of the performance/cost deadlock.
Low Weight & Simplicity. Tilt-rotors of necessity have transmissions crisscrossing the wing: having the engine in the fuselage obviously means transmissions to the tip rotor assemblies; and separate engines in each tip rotor need to be interlocked so that one engine can power both rotors in the event of engine failure. The tip rotor assemblies themselves must be geared to tilt 90 to effect transitions into and out of horizontal flight. All of this means added weight and parts count complexity, with much of the mass located at the wing tips -- the longest moment arm from the fuselage. The wing and airframe in turn need to be stressed to handle these extra loads.
By contrast the Freewing Tilt-Body has simple wing panels, with no transmissions, gearboxes, tip rotor structures. Not only is the Tilt-Body wing structure much lighter than the tilt-rotor's, it can be even lighter than that of a simple fixed wing UAV. This is because the pivoting wing neutralizes turbulent loads against which one otherwise has to design.
Fuel Efficiency. The lower weight of the pivoting wing tilt-body compared to a tilt-rotor means less fuel for a given mission, and weight reduction on both those counts mean that a smaller engine can be chosen for a given design, which in turn means even lower fuel consumption. This is the reverse weight spiral with which aeronautical engineers normally have to contend. As a rule of thumb, it is not unreasonable to expect at least a 50% better ratio of gross takeoff weight versus payload for the Freewing Tilt-Body as compared to a tilt-rotor depending on detail of design.
Ease of Transition. The transition has always been the Achilles' heel of thrust-vectored aircraft. Since such machines tend to be a kind of forced marriage of the helicopter and the fixed wing, while in transition these machines are neither fish nor foul, so to speak. Their aerodynamics are chaotic, and the vehicles are very vulnerable to stalling and falling. Such vehicles are inherently unstable in transition as well as in hover or near-hover. Not surprisingly, this is precisely where accidents tend to occur in conventional thrust-vectored machines.
By contrast the Freewing™ Tilt-Body is inherently stable throughout its flight envelope. Since it is not a fixed wing, but a pivoting wing, it cannot stall during transitions. In an important sense it is true to say that the Tilt-Body at any given instant in time has already completed a successful transition.
Doesn't the tilt-rotor do a full hover, while the Freewing™ Tilt-Body only does a near-hover? Not necessarily. In fact the Scorpion Tilt-Body can fly so slowly that in practical terms it acts the same as a full-hover. In operational terms, even helicopters don't normally hover. They will typically approach for landing at, say, a 45 angle for safety reasons: in case of engine failure they can "autorotate" to a landing. Departures are handled similarly. Therefore most V/STOL missions don't really require full-hover capability. For example, operations from the helipad of a frigate are quite doable by the Freewing™ Tilt-Body. The reason? One can always count on 20 or so knots of wind at sea, even if the ship itself is creating it by being under way. This is enough for the Tilt-Body to hover with regard to the frigate. This is why Matra DÈfense selected the Tilt-Body over all other vehicles it studied, including the Sentinel rotary wing and the Bell tilt-rotor. (Note: Freewing™ has also developed another Tilt-Body, called the Manta, that can fully hover. The company chose to fast-track the Scorpion development because it is simpler than the Manta version, and yet can perform most "VTOL" missions.)
Turbulence sensitivity. The fact that the Tilt-Body automatically neutralizes most turbulence has several important implications for UAV operations, producing advantages compared to tilt-rotors, helicopters and fixed wings.
Image resolution. Image resolution. This after all is what UAVs are all about. Ultimately the customer would not care whether the vehicle itself used antigravity or magic carpet fairy dust. Tilt-rotors, helicopters and fixed wings are all sensitive to turbulence. Acceptable sensor imagery on these bouncing platforms is made possible by mounting the sensor on a gimballed stabilizer. But these stabilizers frequently cost as much as the rest of the airframe combined. Freewing™ can capitalize on this in one of three ways, the choice depending on details of the mission: (i) do without a gimballed stabilizer and still have at least satisfactory imagery since the Tilt-Body vehicle itself serves as a passive first-order-of-magnitude stabilizer; (ii) use a less expensive gimbal system than fixed wings and achieve at least the same image quality; or (iii) use the same stability augmentation as comparable fixed wings, in which case the resulting fineness resolution is greater than is possible with any similar fixed wing. This last would be the choice for such missions as laser target illumination for smart munitions.
Landing near obstacles. This applies to both Army and Navy operations. For the Army that "shoebox" landing area is presumed to be surrounded by trees and have winds up to 20 knots. Since horizontal winds over and around obstacles can create violent mechanical mixing of the air, one has to assume a very gusty environment just at the most vulnerable part of flight, the landing. In the maritime arena matters are, if anything, worse; the air vehicle needs to approach into the wind, which almost by definition has been rendered violent by passing around and over the ship's superstructure. Clearly the pivoting wing's natural alleviation of gusts is a desirable characteristic.
"Mission-capable." Intuitively, it is clear that having a UAV that cannot stall or spin and that is essentially immune to turbulence means that one will be mission-capable in a wider variety of meteorological conditions, even in some cases when conventional technology vehicles are grounded.
C.G. Sensitivity. Aircraft classically are quite sensitive to fore & aft changes in the center of gravity, which means one must be careful how one loads the fuselage for a mission. Fixed wing vehicles typically have safe C.G. ranges no greater than 5 or 10 percent of the mean aerodynamic chord*. But the Freewing Tilt-Body can have an allowable C.G. range in excess of an entire chord length. (This is because torque essentially will not pass through the wing hinge.) That represents an order of magnitude increase in C.G. range.
From a mission-to-mission perspective that is important because the Tilt-Body can accept a variety of payloads much more flexibly, without a correlative reduction in need to redesign or tailor payload packages. This is a significant system cost savings, since sensor packages are expensive to modify.
From a life-of-system point of view, this is important, because as systems mature and "age" and as they experience "mission creep" or the "oh-by-the-way syndrome"** the internal mass must be redistributed to keep pace and to keep the C.G. point appropriately placed. This is a very expensive contributor to an airplanes life-cycle costs. So C.G. insensitivity is a definite cost-savings factor.
Inherent Modularity. This feature of the Freewing Tilt-Body is a significant benefit in terms of (i) portability/stowage, (ii) easy, plug-in field replacement of damaged subassemblies and (iii) mixing and matching wings of varying aspect ratio and area, creating a very inexpensive UAV family. This is not necessarily true of other UAVs. Delta-wings have no such flexibility. More standard fixed wing vehicles can be made more modular, but the "single pin release" that comes naturally to the pivoting wing would come only at a cost and weight penalty to standard fixed wings.
But the modularity of the Freewing Tilt-Body is more profound in its implications than is apparent at first glance, with beneficial nuances impossible for conventional aircraft. For example, one can easily add fuel tank extensions to the inboard "stub wings." These stub wing extensions aerodynamically fuse with the stub wings, increasing the stub wing aspect ratio and adding lift. When used with interchangeable wide span pivoting wings, this provides an extraordinary range and endurance flexibility. Yet the added expense, from a system point of view, is negligible, since the pivoting wings and stub wings are simple structures. The common core is where most of the dollar value is, and that section is untouched.
By contrast, fixed wing UAVs would probably have to add external fuel tanks (adding drag rather than lift) and/or fuselage plugs (extremely expensive).
If the choice is made to re-engine for the largest or longest-range member of this Freewing Tilt-Body family, such a task is a good deal less expensive than the same task for a fixed wing vehicle. The reason? The Tilt-Body is insensitive to C.G. location, while re-engining a fixed wing vehicle would almost certainly involve either adding ballast (penalty weight) to maintain proper C.G., or else redistribute the internal mass (an expensive proposition, as discussed earlier).
Cost savings with the Freewing Tilt-Body. Cost is a major driver in any program, military or civilian. With the Tilt-Body one gets the performance of a tilt-rotor with the simplicity of a fixed wing. Not only is the Tilt-Body dramatically cheaper than other thrust-vectored systems to buy and maintain, as discussed above, but it can be argued that its life-cycle costs may be cheaper even than comparable simple fixed wing UAVs. The logic is in the chart comparison below.
That concludes this briefing.
* The chord is the distance from the leading edge of the wing to the trailing edge of the wing.
** Example: "Oh, by the way, the GPS satellites are now in place; here is a GPS black box you have to retrofit into the vehicle."